Due to a global rise in temperatures, Cambridge will experience longer summers along with higher frequency of heat waves and days with a heat index above 105º.[1] By 2030, heat rise will be an issue, and by 2050, it will be a major problem. In the past, MIT students have not been affected by the summer heat because many students do not reside on campus over the summer, but an extended summer will soon leave student housing in unreasonable conditions: almost every residential building does not have air conditioning (the only exception is New House, which has AC from May to September). A study in Energy Procedia focuses on methods of evaluating heat transfer in building materials.[2] Each dorm should be further evaluated to determine the individual impact of heat on for different buildings. These conditions during heat waves negatively affect both the mental and physical health of students. In addition, energy costs for research buildings will soar over the next decades since temperature in labs must be strictly controlled all day at all times of the year. Creating more energy-efficient cooling/heating systems is crucial for the future–an investment in new technology now is necessary to offset the costs of the huge increase in heat waves in the future.
The main paths toward facing this issue are (1) replacing current systems with well-established and proven energy-efficient energy heating and cooling such as geothermal heating; (2) utilizing experimental cooling systems; and (3) creating campus policies that guarantee students access to air conditioning.
(1) Replace current systems with geothermal heating and cooling.

Figure 1: One central system powered by the Charles River could be used for the entire MIT campus along with the Kendall subway station and other surrounding buildings. Source: National Geographic[3]

Figure 2: Boston University’s geothermal system uses the ground a heat source and sink. Source: Boston University Sustainability[6]
The Energy Efficiency and Renewable Energy team in the U.S. Department of Energy claims that though the installation price is several times that of an air-source system, the additional costs are recouped in energy savings within 5–10 years; system life is estimated at 25 years for the inside components and 50+ years for the ground loop.[9]
Previously successful implementations of geothermal cooling have proven the cost-effectiveness and feasibility of these plans. A case study of the Oregon Institute of Technology reveals the efficacy of geothermal heating. The system has been used since 1964 and currently “heats 11 buildings (600,000 square feet), provides domestic hot water, melts snow on 2300 square feet of sidewalk, and even cools five buildings (277,3000 square feet) during the summer.”[10] This allows savings of around $224,000 each year through heating costs. These energy savings are especially relevant at MIT, in which the cold New England weather would otherwise lead to high heating expenses. Boston University, just across the Charles River from MIT, has implemented this system from 882 through 888 Commonwealth Ave.[6] As summers become hotter, the relative savings actually increase, since the cost of traditional cooling would increase whereas the cost of geothermal cooling would be constant. There are few buildings in the Greater Boston Area that have implemented this technology even though it would be drastically reduce cost and energy use. Another benefit is that the pumps can simultaneously heat or cool different parts of the same building.
(2) Implementing experimental cooling systems
Many groups are currently researching energy-efficient HVAC, and these innovative approaches have great potential to solve the global increase in heat waves over the century. MIT is currently promoting research in this field (see MOFs below), and it can further promote development of these new technologies by piloting them in campus buildings. Since these studies are extremely recent and represent the future of heating and cooling, we should consider experimental technology and its potential role in the distant future:
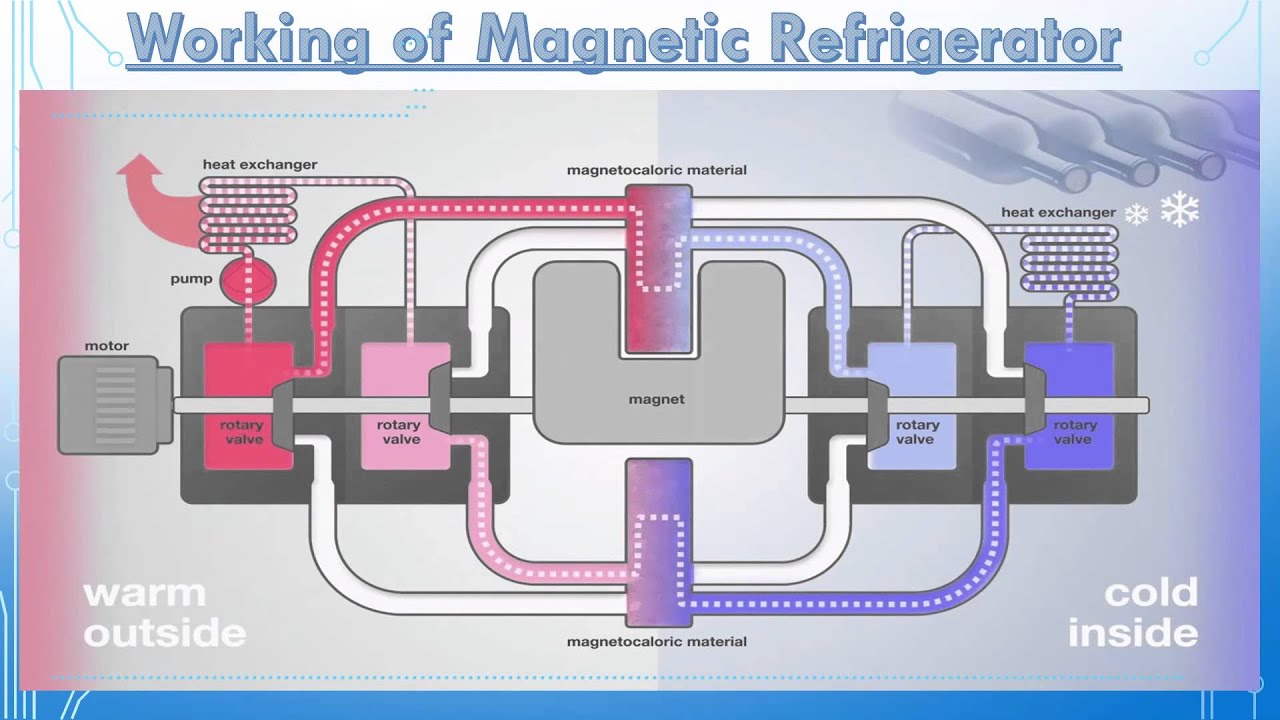
Figure 3: The heat exchange mechanism in a magnetic refrigerator works exactly the same way in air conditioning. Source: BASF[11]
-
- Magnetic air conditioning: study funded by Advanced Research Projects Agency – Energy – U.S. Department of Energy 2010-2014[12]
- Astronautics is currently developing an air conditioning system that is based on the same method of cooling as GE’s magnetic refrigeration. Rather than using liquid refrigerant to absorb heat and pump it into the environment, this new design uses “magnetocaloric materials.” When placed in a magnetic field, these magnetocaloric materials heat up, allowing for heating and cooling in the air conditioning system. This system is more energy efficient than traditional refrigerant-based systems and does not generate the potent greenhouse gases that would otherwise be released by refrigerant.
- Magnetic air conditioning: study funded by Advanced Research Projects Agency – Energy – U.S. Department of Energy 2010-2014[12]
-
- Radiative Sky Cooling: paper in Nature Energy Sept 2017[13]
- A research group from Stanford has created a water cooling system and has started the company SkyCool Systems to commercialize this product.[14] Water coolers sit on the roof where water moves through a tube; the heat then moves a special plastic layer. This layer emits infrared radiation at a wavelength that cannot be absorbed by the atmosphere. Thus, the heat leaves earth into outer space. This approach lowered energy use in the summer by 21%.
- A study on cooling film by Ronggui Yang and Xiaobo Yin of the University of Colorado, in Boulder published in Science Feb 2017 similarly explores the effectiveness of radiative cooling.[15]
- This system is a relatively simple addition onto existing or new air conditioning in buildings and can therefore be combined with almost any other method to further increase energy efficiency with effective cooling.
-
- Metal-organic frameworks: paper published in ACS Central Science Aug 2017[17]
Figure 6: These pores have traditionally been used to store gases, but they could also adsorb water in an air conditioning system. Source: Nature[16]
- Metal-organic frameworks (MOFs) are adsorbent materials with high surface area and pore size. In addition, its structural properties are easily altered. This could allow MOFs to improve adsorption cooling devices. The MIT research group headed by Mircea Dincă is currently studying MOFs and potential uses. Overall, little research has been done on large-scale air conditioning systems (there has been a study on automotive AC) as MOFs have a huge variety of other applications currently being explored.[18] Their moisture-wicking abilities have been studied for humidity control as well as water retrieval in desert environments.[19][20]
These three current experimental air conditioning methods are highly promising and only represent a small sampling of the innovation in the HVAC field. As energy costs due to cooling are projected to increase 10x by 2050 due to global warming, many groups are working on this issue.[14] However, these studies are extremely recent, and none have been implemented on a large scale yet. Though it is important that we follow these studies’ developments over the next decades, at the present, it is uncertain whether these systems will eventually be feasible. Though the increase in heat waves is gradual and may not affect us in the near future, many of these heating/cooling systems are “stackable”: they can be combined to further increase efficiency, and they may be freely added to existing systems rather than completely replacing them.
(3) Policies to guarantee AC for students
For old dorms buildings that do not currently have central air conditioning systems, it may be difficult if not impossible to incorporate new systems. The campus should make AC units more readily available for students in these dorms, either by offering it as an optional addition that is covered by housing costs or simply to make it mandatory for students in the future. The energy costs recouped by use of geothermal heat pumps could fund AC units in dorms in which this technology cannot be applied. This would help offset the additional costs of air conditioning for students who might already have trouble affording tuition and living costs. By 2030, AC should be mandatory for all students–leaving it optional with the onset of more frequent heat waves is too dangerous.
General Plan and Summary:
The ideal solution is to make AC units available for all students immediately and begin installing geothermal heat pumps across the campus. MIT should start with implementing the system in research buildings, whose labs’ consistent need for constant room temperature is a huge energy drain. Since the issue with heat waves and students is not extremely urgent, residential buildings can be more gradually converted, perhaps when some of the experimental systems have been further developed and are ready to be implemented on a large scale. Most of the new innovations in HVAC (heating, ventilation, and air conditioning) can be combined and added on top of an existing air conditioning system, so there is no opportunity cost to choosing a more efficient geothermal system right now. All major changes should be finished before 2050, when heat waves will reach dangerous and costly levels; by then, MIT should have implemented the heating and cooling techniques to keep students cool during the extended summers and to reduce energy costs during all seasons.
By Amy Jin
References
- Massachusetts – States at Risk. (n.d.). Retrieved from http://statesatrisk.org/massachusetts
- N.C. Balaji, Monto Mani, B.V. Venkatarama Reddy. (2014). Discerning Heat Transfer in Building Materials. Energy Procedia 54, 654-668.
- Ten Myths About Geothermal Heating and Cooling – The Great …. (2013). Retrieved from http://energyblog.nationalgeographic.com/2013/09/17/10-myths-about-geothermal-heating-and-cooling/
- Environmental Protection Agency. (1993). Space Conditioning: The Next Frontier (Report 430-R-93-004). Retrieved from https://nepis.epa.gov.
- Geothermal Heat Pumps. (1998). US Department of Energy. Retrieved from https://www.nrel.gov/docs/legosti/fy98/24782.pdf
- Lebovits, Susan Chaityn. (n.d.). Geothermal Building. Boston University. Retrieved from http://www.bu.edu/sustainability/what-were-doing/green-buildings/geothermal/
- Wenisch, J., & Gaunt, L. Inspiring Explorers. (2015). High Performing Buildings, Spring. Retrieved from http://www.hpbmagazine.org/
- Baker, W. F., James, P., Tomlinson, R. F., II, & Weiss, A. (2009). Case Study: Trump International Hotel & Tower. CTBUH, III.
- Geothermal Heat Pumps | Department of Energy. (n.d.). Retrieved November 24, 2017, from https://energy.gov/energysaver/geothermal-heat-pumps
- “NREL: Climate Neutral Research Campuses – Geothermal Energy.” 9 Jun. 2016, https://www.nrel.gov/tech_deployment/climate_neutral/geothermal_energy.html?print. Accessed 27 Nov. 2017.
- Magnetic Refrigeration (A Seminar Video ) – YouTube. (2015). Retrieved from https://www.youtube.com/watch?v=KrQR8h4NKk0
- Air Conditioning with Magnetic Refrigeration. (2010). ARPA-E. Retrieved from https://arpa-e.energy.gov/?q=slick-sheet-project/air-conditioning-magnetic-refrigeration
- Goldstein, Eli & P. Raman, Aaswath & Fan, Shanhui. (2017). Sub-ambient non-evaporative fluid cooling with the sky. Nature Energy 2.
- The Future of Cooling. (n.d.). SkyCool Systems. Retrieved from http://skycoolsystems.com/
- Zhai, Yao & Ma, Yaoguang & David, Sabrina & Zhao, Dongliang & Lou, Runnan & Tan, Gang & Yang, Ronggui & Yin, Xiaobo. (2017). Scalable-manufactured randomized glass-polymer hybrid metamaterial for daytime radiative cooling. Science 355.
- Materials science: The hole story : Nature News & Comment. (2015, April 8). Retrieved from https://www.nature.com/news/materials-science-the-hole-story-1.17274
- Rieth, Adam & Yang, Sungwoo & N. Wang, Evelyn & Dincă, Mircea. (2017). Record Atmospheric Fresh Water Capture and Heat Transfer with a Material Operating at the Water Uptake Reversibility Limit. ACS Central Science 3.
- Baosheng Shi, Raya AL-Dadah, Saad Mahmoud, Ahmed Elsayed, Eman Elsayed. (2016). CPO-27(Ni) metal–organic framework based adsorption system for automotive air conditioning. Applied Thermal Engineering 106, 325-333.
- AbdulHalim, Rasha & M. Bhatt, Prashant & Belmabkhout, Youssef & Shkurenko, Aleksander & Adil, Karim & Barbour, Len & Eddaoudi, Mohamed. (2017). A Fine-Tuned Metal-Organic Framework for Autonomous Indoor Moisture Control. Journal of the American Chemical Society 139.
- Kim, Hyunho & Yang, Sungwoo & R. Rao, Sameer & Narayanan, Shankar & A. Kapustin, Eugene & Furukawa, Hiroyasu & S. Umans, Ari & M. Yaghi, Omar & N. Wang, Evelyn. (2017). Water harvesting from air with metal-organic frameworks powered by natural sunlight. Science 356.